Good evening. Over the last few weeks, I have written several pieces about specific energy technologies (ocean, geothermal, and earlier nuclear). This week is solar power, with particular interest whether crystalline silicon (c-Si) photovoltaic panels will continue to be the dominant solar technology for the foreseeable future, or whether other solar technologies have potential. This will again be an overview piece: I could, and perhaps will, dive much deeper into some specific topics later on.
The most compelling observation about PV technology has been a substantial and persistence price decline.
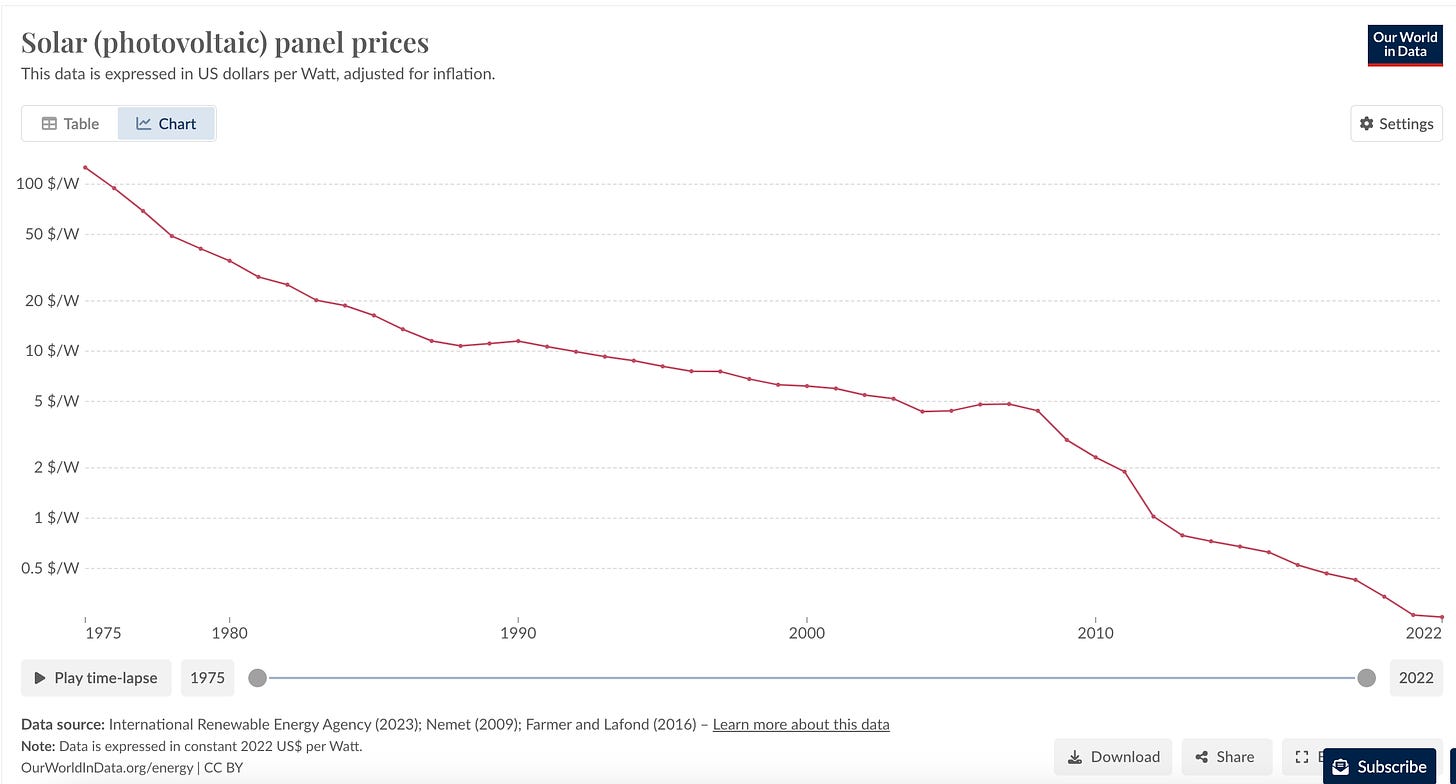
Regularity in the price decline was posited in 2012 and is (falsely) attributed to Richard Swanson and known as Swanson’s law. The principle holds that that the price per watt of solar PV should decline by 20% for every doubling of cumulative manufacturing. Swanson’s law is a form of a learning curve, a concept that dates back to Theodore Wright’s 1936 analysis of the cost of airplanes. These costs declines correspond to rapid growth in the amount of solar PV installed, comprising 4.5% of world electricity generation in 2022.
The causal relation between solar price decline and installed capacity is not as obvious as might appear at first glance, and it is not all obvious that the observed rate of price decline will continue indefinitely. Nevertheless, the price decline is one of the most important trends in the energy industry today. It has also been a major headwind to other low-carbon energy sources, including other forms of solar power.
First off, there are many potential alternatives to solar cells than crystalline silicon. If desired, I would start with this brief explanation of the physics of a solar cell, and then this paper if you want more. Silicon is abundant in the Earth’s crust and has favorable properties for electricity production, and that is why it is preferred.
Thin film solar cells were, in the recent past, an especially appealing solar technology. They have the advantage of potentially lost cost due to reduced material needs and flexibility of deployment, which might make up for the lower efficiency of thin film cells compared to c-Si. As a share of the overall solar PV market, thin film cells have been losing ground since the 1980s, with the exception of a brief resurgence in the 2000s. The market share stands at 5% in 2021, the latest data from the cited report, which is almost the lowest share since 1980.
Thin film cells include cadmium telluride, copper indium gallium selenide, gallium arsenic, and amorphous silicon. Tellurium, gallium, indium, and selenium, unlike silicon, are uncommon elements in the Earth’s crust, which could place limitations on these technologies. Copper zinc tin sulfide (CZTS) may hold promise as an alternative for using nothing but common materials. However, the performance of CZTS lags behind potential, and there are still no commercial CZTS cells on the market.
Perovskite solar cells, named for their crystal structure, have seen remarkable progress in the laboratory in recent years. Their potential efficiency is greater, and manufacturing cost less, than anything that could be achieved with c-Si. There are no commercial perovskite cells on the market today, but there could be soon. One of the persistent challenges is that perovskites degrade when exposed to moisture, and this problem has to be mitigated if they will be commercially successful. Perovskites are a technology to keep an eye on in the coming years.
Quantum dots have long been hailed as “the next big thing” in photovoltaics. The idea of using quantum dots goes back to around 1990. They could potentially have very high efficiency and even generate energy with infrared radiation at night, but they are still far from commercialization. Dye-sensitized solar cells are a related thin film technology with the potential for a low levelized cost of electricity, but so far, low efficiency and stability also confine DSSC to the laboratory.
Organic solar cells are another class that, it is hoped, will feature low manufacturing costs with Earth-abundant materials. As with some other cells mentioned above, efficiency and stability are challenges that prevent organic solar cells from being ready for prime time.
Moving away from photovoltaics completely for now, concentrated solar power (CSP), or solar thermal, is (was?) the other major contender for the solar industry. As the legendary story goes, the use of CSP goes back to 214-212 BC, when Archimedes devised a system to concentrate sunlight with bronze shields and set fire to invading Roman ships. According to critics, there has been little progress since then. However, the CSP industry is still active, with Spain, featuring an ideal climate for it, leading the way. Even more so than PV, CSP functions best in dry, equatorial climates. See this overview from the Solar Energy Industries Association for various CSP methods.
Interest in CSP has waned in recent years, with 2022 showing the first year-over-year contraction. As of 2022, installed capacity was 6.3 gigawatts, which is barely 0.5% of total PV capacity. This is despite CSP levelized costs falling by two-thirds from 2010 to 2021: from 38¢/kWh to 11.8¢/kWh—still too expensive to compete with other mainstream electricity sources.
There is some dispute about whether CSP is truly a “low-carbon” technology, since many earlier projects, such as Ivanpah in Nevada, burned natural gas in the morning to start the plant. This is becoming less of an issue with on-site energy storage becoming the norm.
CSP is still on the Department of Energy’s research agenda, but I find it hard to be optimistic that CSP has much of a future.
There is a way to combine the advantages of PV and CSP, and that is CPV, or concentrated photovoltaics. CPV works by using optics to combine sunlight onto a high efficiency, multi-junction solar cell. The concentration can be as high as 1200 suns or more, meaning that the PV receives 1200+ times as much sunlight as an ordinary unconcentrated panel at high noon on a clear day. At this concentration, the PV needs active tracking and cooling systems.
The rationale of CPV is that, with high concentration, it is economical to use high-efficiency cells. This would improve efficiency and save land compared to ordinary PV.
At this point, a digression into land costs and land use for solar power is appropriate. This paper estimates that a land cost of $80,000 per acre would translate to 1¢/kWh for solar PV costs. In western states, rural land can easily be purchased for a tenth of this price or less. The amount of land required for the lower 48 states to generate all of their electricity from solar PV would be comparable to the land use requirements for other industrial purposes. While land-saving is a laudable goal, it is not a compelling economic driver for more efficient solar production, such as CPV.
Anyway, since the mid-2010s, annual CPV installation has fallen off rapidly, and by now, the industry is just about dead. The problem is that, especially due to the complex tracking systems, it can’t complete with ordinary c-Si PV on cost. There are R&D proposals on improving the geometry of concentrators and other things, and some hand-wavy hope that coproducts such as low-grade industrial heat and greenhouse lighting can sweeten the deal for CPV.
When I started working seriously in energy/environmental policy about a decade ago, CPV is a technology that I had high hopes for. Now, I find it very difficult to be optimistic.
A luminescent solar concentrator (LSC), also called building-integrated solar, is a device that would collect sunlight in a waveguide and direct it toward smaller PV collectors. Like CPV, LSC would concentrate sunlight well above 1 sun, though it wouldn’t get near the extreme levels that contemporary CPV research has achieved. One advantage to LSC is that it would work better than conventional solar on cloudy days and at odd angles, which would make it ideal for building integration. Colorful images like this one help stimulate interest in LSC.
LSC is in a pre-commercial phase, meaning that there are private companies working to bring it to market, but they have not done so yet. There is still fundamental research required to bring LSC from its current best efficiency (~7.6%) to the 30+% that is hoped for. Work on the waveguide material and luminosphores, the materials that absorb and re-emit light, is needed.
There are several potential applications beyond electricity production, and LSC has some promise. But it will be some time before it are ready for the market.
Now, on the subject of truly obscure solar technologies, there is the solar updraft tower. The idea is pretty simple. Concentrators direct sunlight to the base of a large chimney, which heats the air at the base. Since hot air rises, the rushing air can then power wind turbines.
There have been a few prototype experiments, most notably at Manzanares, Spain. That experimental plant entered service in 1982 and collapsed in a dust storm a few years later. Some studies have found that a solar chimney could be economically viable; I’ll believe it when I see it.
And finally, we come to space-based solar power (SBSP). An SBSP system would work by generating power in geosynchronous PV satellites, which would convert the power to microwaves and send it to a ground-based rectanna. SBSP would be more land-efficient than ordinary solar, and the satellites would function almost 24 hours a day, solving the intermittency problem.
Estimates on how much an SBSP system would cost are all over the map, and this is a topic that I would love to write about in more detail later. John Mankins is a strong believer in SBSP and envisions architectures by which it would work. Accounting for the forthcoming Starship system in development by SpaceX, which should further greatly reduce launch costs, a recent NASA report estimates that SBSP would cost 12-80 times as much as other renewable energy sources.
Another hope is to bypass launch costs by using self-assembling factories on the moon to manufacture solar satellites, and from there to launch them into geosynchronous orbit.
Despite the long odds, research is ongoing. CalTech, for instance, recently demonstrated wireless power transmission.
Perhaps at some distant future date, when space launch with Lofstrom loops or orbital rings is routine, launch costs will be low enough so that SBSP becomes routine. A civilization that could do such a thing probably has many other energy options available, such as fusion. More realistically, I imagine that SBSP could be a good option for space settlements, which have few other good options, but I have a hard time imagining that SBSP will be common on Earth.
Fun fact: many people first heard about SBSP from SimCity 2000, in which such plants (microwave plants) become available around 2020, which at the time was well into the future. In the game, it was possible for the beam to miss and cause a major disaster. In real life, this would not be possible.
OK, that’s a lot of solar stuff. There are indeed many possibilities beyond the standard c-Si panels that we know. But for the foreseeable future, I expect c-Si to be the dominant solar technology, with no alternative gaining a foothold in the market. This is because c-Si has a major advantage of well-established supply chains and an active industry that induces learning curves.
Eventually, the cost reductions for c-Si will grind to a halt. This is inevitable, though so far, predictions about an end to cost declines have proven premature. When that day comes, there will be a wider opening for thin film and advanced solar cells, LSC, and maybe even for CPV to make a comeback or for some of the more exotic options. Despite the dominance of c-Si today, it would be a mistake to take a triumphalist attitude by imagining that the energy challenge has been solved once and for all.
Quick Hits
Last night, many of us were treated to an especially low latitude appearance of the aurora borealis (aurora australis in the southern hemisphere), thanks to the strongest solar storm in many years. It was my first opportunity to see the northern lights in person. There are some reports of damage.
I regularly read, or at least skim, Andrew Ng’s The Batch on machine learning. His latest column notes that last year’s sensationalist fears about risks from artificial intelligence has greatly subsided, hopefully creating an opening for a more sensible regulatory response.
Eli Dourado’s recent piece about social collapse, drawing heavily on the work of Joseph Tainter, is worth reading. Dourado suggests that the prevalence of certain nihilistic political movements could be a sign of public detachment from the Western civilizational project. I find this interesting but not convincing, mainly since useful historical context for today’s movements is lacking.
I think far more relevant is when do the cost declines in the TIC flatten. Now that panels are only a small fraction of total cost, things like labour, supports, wires, inverters etc become far more relevant to the actual costs, and we have far less reason to expect the same sorts of learning rates as factory produced modules.
We are seeing the same in grid battery systems, where cell proces are dropping quickly, but announced projects are stubbornly high.