Last week, I discussed transportation of carbon dioxide, particularly with CO₂ pipelines. Transportation is one of several components of the CCUS (carbon capture, use, and sequestration) process, which could be summarized as follows.
Capture of CO₂, either from industrial point sources or the ambient atmosphere.
Transportation of CO₂ from the point of capture to the point of use or disposition.
Use or sequestration.
It was not my intention last week to make this a series, and thus cover the steps out of order, but that’s how it is turning out. This week will be an overview of carbon reuse, and next week may or may not be a discussion of CO₂ capture. We’ll take a brief look at some of the major reuse options.
Electrolyzed Methane
I discussed electrofuels a few months ago, and I won’t belabor the point that most synthetic fuels generated from electrolysis are likely to be infeasibly expensive for the foreseeable future.
Beyond cheap electricity for electrolysis, carbon-based electrofuels require a source of carbon. Such fuels include methane, methanol, and complex hydrocarbons such as kerosene for jet fuel.
Methane, chemically CH₄, is the primary component of natural gas and is used widely as an energy source for electrical power, heating, and various industrial processes. Because natural gas is so cheap and abundant, there is not much incentive to produce methane industrially. The only such facility in the United States is the Great Plains Synfuels Plant, in North Dakota, which operates by lignite coal gasification via the Fischer-Tropsch process. The plant opened in 1984, shortly before natural prices fell, yet continues to operate.
But we are more interested in the Sabatier process for producing methane. The chemical formula is
CO₂ + 4H₂ → CH₄ + 2H₂O
If the hydrogen can be produced from low-carbon means and the CO₂ can be captured from an industrial source or the atmosphere, then the Sabatier process is a lower carbon process for producing methane.
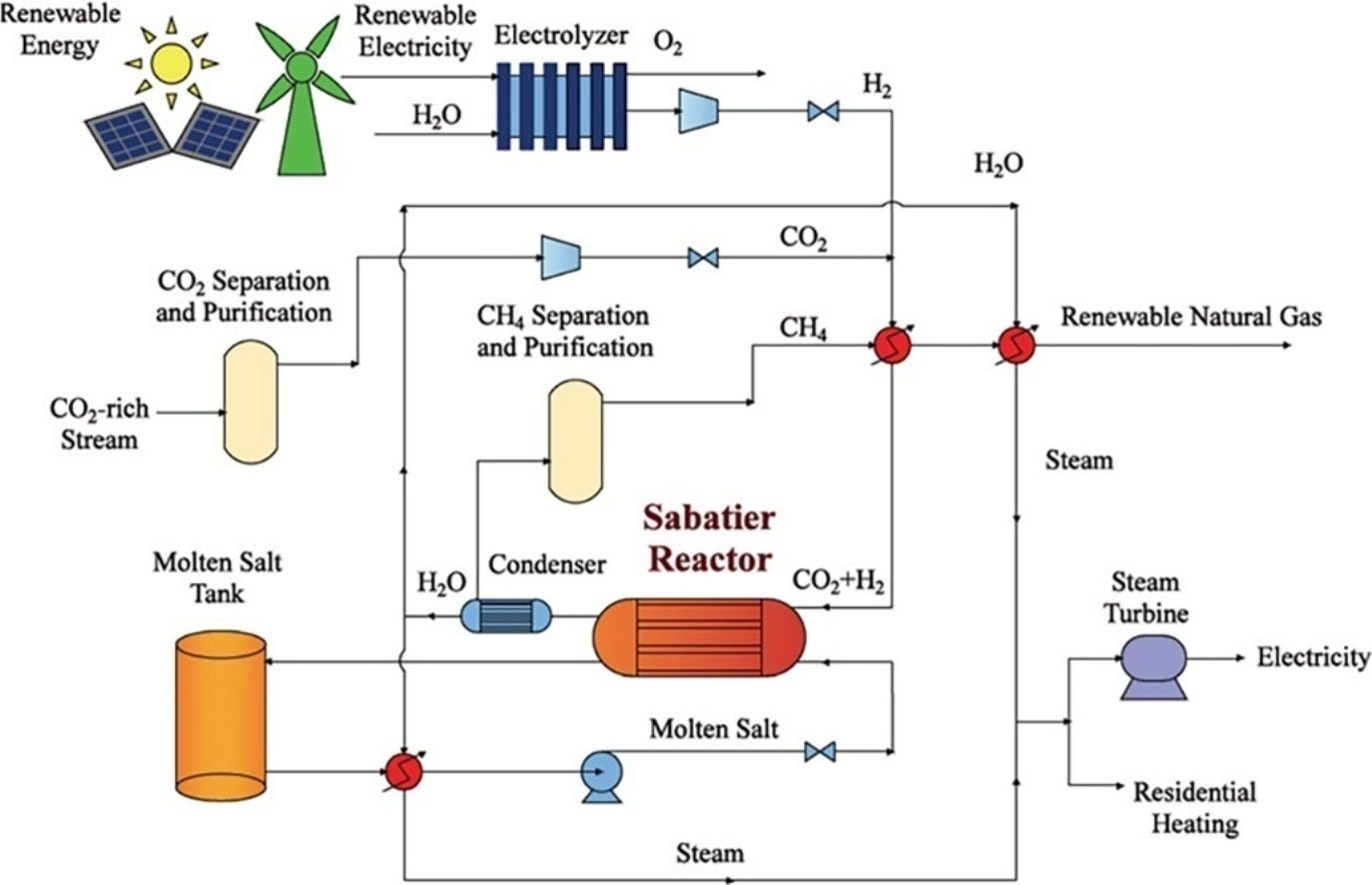
Notice that I said lower, not low, carbon. If CO₂ is sourced from industrial carbon capture, then we essentially have a single CO₂ molecule emitted to the atmosphere over two process: whatever the CO₂ was captured from, and then from the methane combustion. It only becomes a truly low-carbon process if the CO₂ is sourced from the atmosphere via direct air capture, or if carbon capture can be applied again and again to each combustion until it is sequestered for good.
As I discussed before, this process is way too expensive to be practical on an industrial scale today. Today, the main use of the Sabatier process is to produce water, rather than methane, on the International Space Station. The process is also of interest to manufacture rocket fuel on Mars for a future human mission.
Based on a chemical mass balance, one ton of CO₂ should produce about 0.37 tons of CH₄, minus any conversion inefficiencies. About half of world CO₂ emissions from energy come from point sources, so let’s say that’s about 20 billion tons per year, which could produce about 7 billion tons per year of methane. World natural gas production is about 145 billion cubic feet. If I did all my conversions correctly, that should be about 2.6 billion tons, and so it would appear that electrolyzed methane, though not sufficient to absorb all possible CO₂ capture, should be more than enough for the foreseeable future.
Except, for reasons of basic thermodynamics, a world in which it makes sense to electrolyze methane with the Sabatier process is not a world in which it makes sense to burn natural gas for electricity. That would be like trying to power a light bulb with a solar panel, whereby the solar panel is powered by shining the light bulb on it. In a world of electricity abundant enough to power the Sabatier process, heat and most industrial processes using methane have probably been electrified. And so the realistic market for the Sabatier process on earth, should it ever become economically feasible, is almost certainly much smaller.
Methanol
I discussed methanol a few weeks ago in the context of electrofuels, but methanol is a highly versatile industrial chemical. Chemically CH₃OH, or also MeOH, methanol is like methane, except one of the hydrogen atoms is replaced by hydroxide. World methanol production is about 110 million tons per year, and if I did my math correctly, it should require about 150 million tons of CO₂ per year. That’s a bit less than 1% of my back-of-the-envelope figure above for the amount of point-source CO₂ that would be available.
The largest and first commercial methanol plant to use recycled CO₂ is the Shunli plant in Anyang, China, based on technology from Carbon Recycling International, which came online in 2022. The plant can process up to 160 thousand tons of captured CO₂ per year. It’s a drop in the bucket compared to overall emissions, but that’s where a nascent industry starts.
Jet Fuel
Electrolyzed jet fuel is of particular interest because long-haul aircraft cannot be electrified directly with any foreseeable technology (though there are some interesting goings-on with flywheels using exotic materials; maybe I’ll write about that some day). Most conversation about low-carbon aviation revolves around sustainable aviation fuel, a biofuel that can be blended into petroleum-based jet fuel. SAF raises similar land use questions that are posed by corn-based ethanol for blending into gasoline, such as competition with the food supply and land use change, and so we can ask how “sustainable” it really is.
If I am doing my calculations correctly, world jet fuel demand in 2023 was about 90 million tons. Taking kerosene as C₁₂H₂₆, this would require around 280 million tons of CO₂. This report assesses that about a billion tons could be captured per year from 2022 to 2050; I’m guessing the difference is, at least in part, due to anticipated growth in aviation over the coming years.
Although the economics are not very favorable, there is plenty of room for improvement because the technology is nascent. A paper this year, for instance, finds that world power-to-kerosene efficiency for current facilities is about 39% and could improve to 54%. In 2023, a company called Twelve opened what I believe is the world’s first electrolyzed jet fuel plant.
Olefins
Olefins are a class of chemicals composed of carbon and hydrogen with one or more double-bonds between carbon atoms. They are widely used in polymer products, including many plastics. The two leading classes of olefins—ethylene and propylene—had annual productions of 150 million and 80 million tons per year respectively a few years ago. That might be a market potential of around 1.1 billion tons CO₂ per year, based on 4.88 kg CO₂ required for 1 kg of olefins.
There are some ongoing research projects, such as this one and this one. I am not aware of any commercial facilities. This recent paper finds that a power-to-olefins system would save 47% CO₂ emissions relative to traditional fossil-based production when powered by a low-carbon energy source—disappointing, I know. The process would also cost about three times as much as the market price, which is in line with the economics of various electrofuel routes.
Other Uses
Enhanced oil recovery refers to several different techniques, one of which is injecting carbon dioxide into an oil well to decrease oil viscosity and thus increase production. EOR might not be our favorite reuse of CO₂, but it is the most prevalent. As of 2011, EOR constitute about two-thirds of CO₂ reuse in the United States at 50 million tons per year.
This paper finds a potential of 3 billion tons per year of CO₂ sequestration in building materials, “binder systems involving Portland cement, non-hydraulic calcium silicate, industrial solid wastes and magnesium-based materials”. Among the startups in this space include the British firm Seratech, which is developing a magnesium carbonate binder for bricks that uses captured CO₂. This paper finds a potential for captured carbon for concrete to sequester 100 million to 1.4 billion tons, but notes that overall lifecycle benefits may be considerably less.
The FAO finds that world algae production was 35.82 million tons in 2019 and growing rapidly. Since updake of CO₂ by algae is about twice the weight of the algae, that should be a market potential of about 70 million tons. The Algae Biomass Organization highlights some commercial and research efforts, but I am not aware of any commercial facility that has shown commercial maturity.
Certain advanced carbon-based materials, such as carbon nanotubes, might be produced with captured CO₂. The CNT market is growing fast but from a tiny base; this paper forecasts 7000 tons in 2025. The greater relevance for carbon-based nanomaterials, such as graphene, is their possible applications in capture.
Carbon dioxide is what is used in beverage carbonation. A can of Coca-Cola has 2.2 grams of CO₂, and so if everyone in the world drank a can of Coke every day, that would be about 6.4 million tons of CO₂ sequestered per year. Also, that popping sound you hear when you open a can of soda is the sound of CO₂ being un-sequestered.
Conclusion
Here I have tried to survey major potential CO₂ reuses, with an emphasis on estimating the volume of carbon dioxide that could be captured and used. These estimates are rough and ready and I hope at least within an order of magnitude of being right. I have tried to survey all the major proposed carbon reuses, and a few minor ones, but I may have missed some. I have also looked mostly as theoretical limits to how much CO₂ could be used; actual numbers will certainly be much less when we account for various technical, economic, and political constraints.
Sequestration is not a particularly satisfying solution to CO₂ emissions for as long as reuse remains on the table. As I hope this review has shown, reuse opportunities, though not infinite, are substantial and will be enough to absorb the supply of captured carbon for the foreseeable future and perhaps forever.
Unfortunately, economic constraints, rather than overall market capacity, is the major barrier to growth here. As I’ve discussed with electrofuels and olefins, the CO₂ reuse routes are substantially more expensive than the conventional routes, and as long as this remains true, reuse cannot be more than a bit player.
I should say this explicitly more often, but I am always grateful to hear thoughts, personal experiences, corrections, and friendly disagreements on the topics. Did I miss any important reuse options? Am I overly optimistic or overly pessimistic about reuse economics? If I were to continue writing about CC(U)S, future topics will be the capture costs from various industrial sources; direct air capture; geologic sequestration; and the political economy of CCUS, including whether carbon capture prolongs the usage of fossil fuels and whether this is a problem.
Quick Hits
The Economist reports that, after years of dazzling progress in poverty reduction around the world, especially since 1990, poverty reduction and economic development in low-income countries have nearly ground to a halt since 2015.
Is the sunk cost fallacy really a fallacy? If we undertake an endeavor, and then find that the endeavor is more costly, or the reward less valuable, then initially assessed, then it is rational to abandon that endeavor if the remaining cost is assessed to exceed the reward. The trouble with many logical fallacies is that they assume that a person is able to assess the situation with generally reliable accuracy. The sunk cost fallacy—the tendency to plod along after an endeavor has been undertaken—may in fact be a rational deference to our past judgment in the face of our inability to accurately assess costs and benefits.
The Cosmos Institute is a new think tank about the role of artificial intelligence in society. Their work is a bit airy for my tastes, but I’ll be curious to see what comes of it. I am thinking of participating in their essay contest, and if I do, next week’s post will be short.
I have enjoyed this series, keep up the good work 👍
> The Economist reports that, after years of dazzling progress in poverty reduction around the world, especially since 1990, poverty reduction and economic development in low-income countries have nearly ground to a halt since 2015
The poverty reduction in Asia was due to their industrialisation revolution. The growth in Africa was mostly a commodity cycle that's unlikely to come back. I'm oversimplifying here but that's the gist of it.